Why thermal conductivity matters — and gel based products fail
Increased thermal conductivity allows for a faster rate of heat transfer in phase change material, reducing the time required for the PCM to undergo a complete charge or discharge.
By Aymara Albury, Ph.D.
Thermal conductivity is the intrinsic property of a material that expresses its ability to conduct heat. It is often denoted as κ, k or λ. Thermal conductivity is measured in watts per meter-kelvin (W/(m·K)) and is therefore a rate measurement of heat flow, through a material of a given thickness, across a surface area perpendicular to the source, resulting from a temperature gradient (Figure 1). Two techniques are used to measure thermal conductivity: steady state and transient. For steady state methods, thermal conductivity is determined once the sample reaches a temperature equilibrium and a constant signal is generated. Transient (non-steady state) methods allow for thermal conductivity to be measured as the sample is being heated.
Phase change materials (PCMs) store and release energy when they transition between their solid and liquid states. This energy is in the form of latent heat. Thermal energy must flow either into or out of the PCM in order for the phase change to occur. Therefore, the time required for a PCM to completely charge or discharge is directly dependent on its thermal conductivity. In addition to increasing the thermal conductivity of a PCM, there are several ways to increase the rate at which a PCM melts and freezes. These include increasing the turbulence of the heat transfer fluid, increasing the surface area that the heat transfer fluid has contact with and increasing the temperature gradient (ΔT) between the PCM and heat transfer fluid.
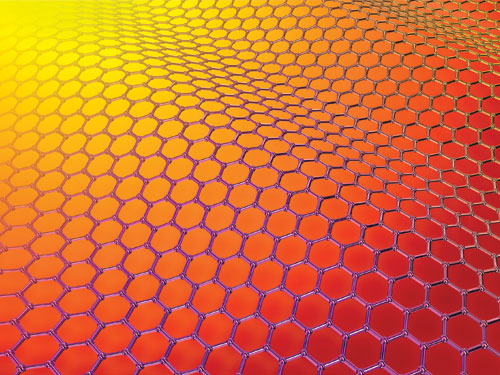
Thermal conductivity varies depending on the nature of the material. Metals, which primarily use electrons for heat transfer, tend to have higher thermal conductivity values. Non-metals, which use phonons for heat transfer, have lower ones. Organic PCMs are non-metal compounds that exhibit very low thermal conductivity values. This property presents a challenge when bio-based PCMs are used in applications that require rapid charge and discharge.
Thermally conductive additives have been explored in an effort to improve the overall thermal conductivity of such PCMs. Additives such as expanded graphite, aluminum, copper and aluminum nitride can increase a PCM’s overall net thermal conductivity and allow for a faster rate of heat transfer. This can reduce the time required for the PCM to undergo a complete charge or discharge.
Another approach that can be taken to minimize the adverse effect of thermal conductivity is using containment options that increase the surface area and reduce the distance from the external heat source to the center of the PCM. Increasing the surface area that the heat load can be applied to and reducing the distance that the heat has to travel will increase the likelihood that the PCM will undergo a complete charge or discharge in a shorter period of time.
Increasing the turbulence of the heat transfer fluid increases the rate of heat transfer between the fluid and the PCM, thereby improving the rate of melting and freezing. The additional turbulence disrupts the insulating blanket that is formed between the PCM container and heat transfer fluid as a result of laminar flow. In some applications, an increase in turbulence for the heat transfer fluid is not possible.
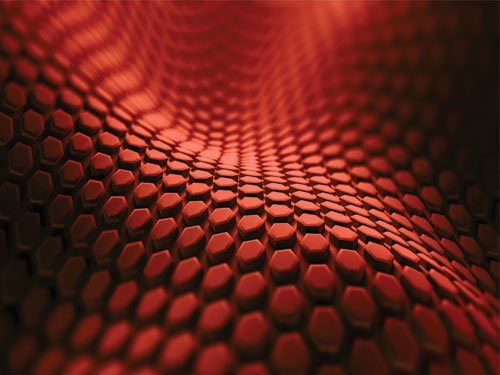
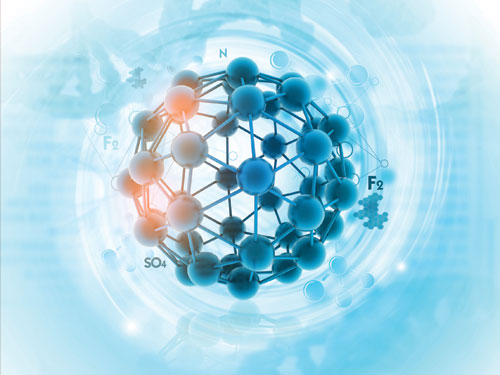
The temperature gradient also plays a significant role in the rate of PCM melting and freezing. The larger the ΔT, the faster the rate of heat transfer between the PCM and heat transfer fluid, which causes the PCM to melt or freeze faster. However, when the ΔT is small, the rate of heat transfer is significantly reduced, which slows down the melting and freezing process. In most PCM applications, however, the temperature gradient between the PCM and heat transfer material cannot be manipulated to improve the rate of melting and freezing.
About the author
Dr. Aymara Albury joined Entropy Solutions in 2013 after earning her Ph.D. in synthetic organic chemistry from the University of Alabama. She earned a Bachelor of Science degree in chemistry at Alabama in 2007.